Physicists shine light on properties of potential solar cell material
Oct 7, 2019 08:43 PM ET
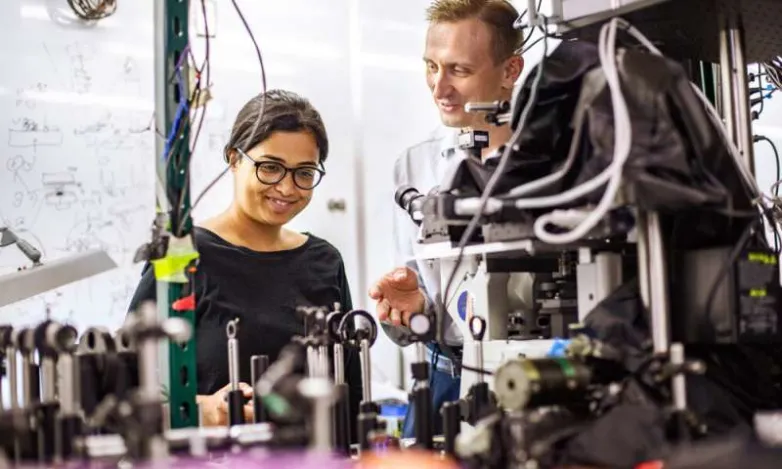
Research led by University of Texas at Dallas physicists has altered the understanding of the fundamental properties of perovskite crystals, a class of materials with great potential as solar cells and light emitters.
Published in July in Nature Communications, the study presents evidence that questions existing models of the behavior of perovskites on the quantum level.
"Our enhanced understanding of the physics of perovskites will help determine how they are best used," said Dr. Anton Malko, associate professor of physics in the School of Natural Sciences and Mathematics and a corresponding author of the paper.
The term perovskite refers broadly to minerals with the same specific crystal structure as the compound that originally bore the name perovskite: calcium titanate.
"In any pure crystal, atoms are arranged in a very orderly fashion," said Dr. Riya Bose, postdoctoral research associate in physics who prepared the samples for the study. "Thousands of materials can be defined as perovskites by their particular structure. Certain types of these are excellent candidates for solar cells or light emitters."
Perovskite research is relatively young, beginning with photovoltaic studies published about a decade ago. By comparison, silicon solar cells have been refined for many decades.
"Silicon solar cells, like those you can buy now, have become more efficient over the decades, increasing dramatically after the 1960s," Malko said. "Today's efficiency is at about 20 percent," meaning that one-fifth of the energy of the incident light is converted to electricity by solar cells.
Because perovskites are a newer research topic, much remains unknown about why they behave as they do.
"What is known, however, is that perovskites already exceed the top efficiency achieved with silicon," Malko said. "They are also easy to make and are very inexpensive relative to silicon."
What holds perovskite back is its instability; a perovskite solar cell would need to be replaced in a matter of weeks.
"A solar cell that degrades rapidly under sunlight is clearly useless," Malko said. "A silicon cell could last 20 years. With perovskite, life spans are measured in hundreds of hours. But even that represents progress from the earliest research. Now, we have moved to working with inorganic perovskites, which should extend that life."
Many materials with good light absorption rates also are good at reemitting that light. Malko's work has focused on the light-emitting behavior of perovskites at the nanoparticle level.
"The quantum yield for some perovskite particles is nearly 100%, which means they're super bright," he said. "We set out to find the specific source of this luminescence."
Before Malko's study, the widespread model was that within perovskites, as in many other semiconductors, light is emitted by excitons: bound states of negative and positive charges, respectively called electrons and holes. Excitons can move over wide distances within the material.
According to this model, as the size of the material shrinks, the excitons should become more restricted in their movement, a process called quantum confinement. This should result in changes in the wavelength, or color, of light absorbed or emitted.
Using single particle spectroscopy to observe perovskite nanoparticles, the scientists wanted to learn what individual excitons were doing. In testing the conventional wisdom, they disproved it.
"We observed that perovskite light is remarkably consistent," Malko said. "Despite examining a wide range of sizes, from 9 to 30 nanometers, the emission wavelength—the color of the light—was unchanged in the cesium-based perovskite samples," he said. "The emitted light was a specific green no matter the size of the material observed."
What Malko, Bose and their colleagues found—both in perovskites that possess internal three-dimensional crystal lattices and in zero-dimensional ones—was that the light emission on the single nanoparticle level looked more like light from individual, strongly localized molecular excitations rather than from mobile electrons and holes. Digging further, the researchers determined that the source of the emitted light was tied closely to the bromide atom vacancy sites within the perovskites.
"These findings contradict the quantum confinement model, which would dictate that the source of luminescence in these perovskites is from excitons delocalized over nanoparticles," Malko said. "Perovskites of any size will demonstrate this behavior."
Additionally, in a case of quantum confinement, exposure to more intense light would create more excitons with different behavior and emissive properties. In the cesium-based perovskite nanoparticles, however, enhanced photon output was characterized by similar emission parameters.
"This is dramatically different from the previous views of the community," Malko said. "The prevailing preconception involving quantum confinement has been difficult to displace."
Malko described the research as an important step forward in understanding the emission properties of perovskite materials. Still, a number of issues need to be resolved before their practical implementation—chiefly, the longevity problem.
"If someone finds a way to make perovskites last for several years, I predict there will be dozens of companies making perovskite solar cells and light-emitting devices," he said.
Also read
- Breakthrough in 23.2% Efficient Low Band Gap Solar Cells
- CityUHK Secures Funding for Advanced Solar Cell Research
- UtmoLight Unveils 450W Perovskite Solar Module Breakthrough
- Microquanta Achieves Record 23.65% Efficiency in Perovskite Modules
- Breakthrough Method Achieves 28.2% Efficiency in Tandem Solar Cells
SOLAR DIRECTORY
Solar Installers, Manufacturers